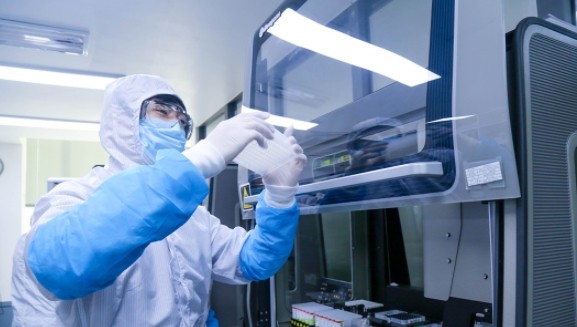
Abstract
Objectives:
Healthcare surfaces contribute to nosocomial disease transmission. Studies show that despite standard guidelines and practices for cleaning and disinfection, secondary infection spread among healthcare workers and patients is common in ICUs. Manual terminal cleaning practices in healthcare are subject to highly variable results due to differences in training, compliance, and other inherent complexities. Standard cleaning practices combined with no-touch disinfecting technologies, however, may significantly lower nosocomial infection rates. The objective of this study was to evaluate the efficacy of a whole-room, no-touch disinfection intervention to reduce the concentration and cross-contamination of surface bacteria when used in tandem with manual cleaning protocols.
Design:
Bacterial tracers were seeded onto hospital room surfaces to quantitatively evaluate the efficacy of manual terminal cleaning practices alone and in tandem with a no-touch, whole-room atomization system. Cross-contamination potentials and labor efficiency were also evaluated.
Subjects and Intervention:
Environmental service personnel cleaning efficacy was evaluated pre and post application of manual terminal cleaning protocols alone and in tandem with a whole-room atomization system with an United States Environmental Protection Agency-registered hospital-grade hypochlorous acid disinfectant.
Setting:
The study was conducted in an unoccupied patient room at Banner University Medical Center in Tucson, AZ. The room was located in a newly constructed ICU suite.
Measurements and Main Results:
Manual terminal cleaning averaged a 2.4 log10 reduction in seeded bacterial counts compared with a 4.9 average and up to a 6 log10 reduction with tandem cleaning. Cross-contamination among surfaces following terminal cleaning alone was documented in 50% of the samples compared with 0% with tandem cleaning, with the latter achieving a 64% improvement in manual labor efficiency.
Conclusions:
The use of whole-room atomized disinfection with terminal cleaning protocols lowered manual labor times, improved disinfection outcomes, and eliminated the transfer of bacterial pathogens in healthcare environments.
Environmental surfaces contribute significantly to the transmission of pathogens in healthcare environments (1,2). Despite the availability of federal and facility-specific guidelines for cleaning and disinfecting patient rooms (3), evidence suggests that pathogens, including drug-resistant bacteria, persist post cleaning and are transmitted to other patients (4,5) and personnel (6,7). At least eight studies have shown an average 120% increased risk of acquiring a bacterial healthcare-associated infection (HAI) if the room was previously occupied by a colonized or infected patient (8,9). A meta-analysis of 33,494 patients found that patients who occupied an ICU bed whose prior occupant carried a bacterial pathogen were up to 8.64 times more at risk of acquiring the same pathogen (10). These secondary infections provide evidence of transmission via the environment (11).
Manual cleaning efficacies are highly variable and may be impacted by staff education and training, workflow issues, compliance with disinfecting protocols, and more (12). Terminal cleaning is often suboptimal, and up to 56% of surfaces are inadequately cleaned following patient discharges (13,14). Interventions focused on optimization of cleaning protocols, environmental service worker education, and increased monitoring have successfully increased cleaning efficacy, but such programs are challenging to sustain (15).
No-touch technologies, including cleaning robots, have gained in popularity and have been shown to reduce microbial surface contamination, positively affect clinical outcomes, and add an incremental benefit to standard manual cleaning (16). Although not a replacement for manual, physical cleaning, when used in tandem, whole-room disinfection systems may have an added benefit. Few studies have evaluated the benefits or sustainability of routine use of no-touch disinfection systems for reducing HAIs in hospitals. Others have reviewed clinical evidence for no-touch methods, including ultraviolet light devices and hydrogen peroxide systems for terminal room decontamination (17,18). Results indicate that such devices can provide supplemental benefits for the increased inactivation of bacteria and viruses on hospital room surfaces and subsequently reduce patient colonization and HAI rates. The emergence of antibiotic-resistant bacteria and associated ICU outbreaks continues to be reported (19,20); however, the use of enhanced surface disinfection protocols has been shown to reduce infections in these complex environments (21,22).
This study compares the efficacy of manual terminal cleaning practices alone and in tandem with a whole-room, hypochlorous acid (HOCl) atomizing disinfection system for the reduction of bacterial tracers seeded on selected hospital room surfaces. Also, we quantified the spread of bacteria during each cleaning practice from one seeded site in the room to other nonseeded sites.
MATERIALS AND METHODS
Sample Location
The study was conducted at Banner University Medical Center in Tucson, AZ. The room was located in a newly constructed ICU suite within a nine-story tower. The unoccupied patient room was set up to resemble an active hospital room representative of other patient rooms in the facility and included a hospital bed, bedside table, IV pole, two chairs, and various electronic devices (i.e., phone, ultrasound machine). This study did not meet the definition of human subjects research and thus was not submitted for further review. The study protocol was reviewed and determined to be safe by The University of Arizona’s Research Laboratory and Safety Services Biosafety Committee.
Whole-Room Atomizing Disinfection
The efficacy of tandem, whole-room atomized disinfection was evaluated using a Nimbus mobile unit (Nevoa, Tempe, AZ). The operation of the whole-room atomization system was performed by a trained member of the Nevoa team under third-party supervision. Before use, room air vents and smoke detectors were sealed using temporary covers, and the external, self-sealing door was closed to prevent the escape of the disinfectant fog. After the room was sealed, a series of automated steps were initiated by the user from a wireless tablet computer located outside of the room. Next, the unit automatically completed a series of steps. The first step was to atomize a United States Environmental Protection Agency (EPA)-registered hospital-grade disinfectant containing HOCl as the active ingredient approved for use in fogging, atomizing, or aerosol applications (Nevoa Microburst Solution, Nevoa, Tempe, AZ; EPA registration number NLS-90880-1). After completing the atomization step, the unit automatically transitioned to a dehumidification step. The complete cycle collectively ran for 31 minutes, achieving a minimum required 10 min disinfectant contact time. This cycle duration was preprogrammed based upon the room volume of 2,392 cubic feet.
Traditional Terminal Cleaning
Terminal cleaning was coordinated with the hospital infection preventionist and the environmental service staff (EVS). Cleaning was conducted during each trial by a trained member of the hospital EVS team who was familiar with the hospital’s standard, approved cleaning protocol. This protocol included a full wipe down of all surfaces, equipment, and walls using a hospital-grade disinfectant, with special attention to the bed, bedside area, and bathroom. Following the surface wipe down, the floor was mopped with a cleaning solution starting from the back of the room and progressing toward the door.
Terminal Cleaning + Whole-Room Atomized Disinfection (Tandem Cleaning)
Terminal cleaning plus whole-room atomized disinfection was implemented using a modified traditional cleaning protocol. This included a full wipe down of the bathroom, sink, and door handles with a shortened wipe down of the bed and bedside area. The walls, visitor furniture, counters, and other horizontal surfaces were not manually wiped down. Immediately following this wipe down, the whole-room atomized disinfection unit was brought in and allowed to run according to the above protocol. Once the unit finished its cycle, it was removed, and the floor was mopped from the back of the room toward the door.
Sample Collection
A total of 14 surfaces representing 12 high and two low touch items were seeded with 100 µL of Escherichia coli (ATCC 15597) at a concentration of 108 colony-forming units (CFUs)/mL. E. coli was chosen as a model organism for other bacterial pathogens due to its safety factor for field use, extensive use in other studies, and ease of culturing. Each surface was seeded in two adjacent locations. Following a 30-minute drying time, one of the seeded locations on each surface was swabbed using 3M swabs containing 1 mL of letheen disinfectant neutralizing broth (3M, Maplewood, MN). Following sample collection, the room was disinfected with either traditional terminal cleaning or modified terminal cleaning plus whole-room atomized disinfection (tandem cleaning). After the cleaning was completed, the second location of each seeded surface was swabbed. Samples were kept on ice and brought to the laboratory for processing. The nature of this study was hypothesis generating, and sample size was selected based on convenience of data collection and in consideration of time and cost limits. Each trial was repeated four times on separate days for a total of 8 test days and 112 samples per cleaning protocol (56 pre and 56 post cleaning). Following sample collection, each site was disinfected with 70% ethanol to eliminate any residual seed concentrations.
In addition to seeded sample collection, a subset of samples was collected 30.5 cm away from the seeded location for each cleaning method (terminal and tandem) for a total of 16 samples per cleaning protocol. This cross-contamination study was used to determine if any microbial spread occurred from seeded to adjacent sites during the implementation of terminal compared with tandem cleaning methods. Targeted locations to evaluate cross-contamination included chairs, bed rails, floors, and bedside tables. The 30.5 cm distance was selected based on the size limitations of the smallest targeted surface (bedside table). Recently, others have documented cross-contamination potentials from seeded sites to nearby adjacent surfaces and up to 2 m2 during the use of disinfecting wipes (23). These complete cross-contamination trials were repeated in triplicate.
Sample Assays
To maintain an unbiased assessment, field samples were blinded and assayed in the laboratory by personnel who were not part of the field collection team. All samples were diluted and cultured onto trypticase soy agar (BD, Franklin Lakes, NJ) using the spread plate method. Samples were incubated at 37°C for 24 hours before enumeration. Concentrations were determined, and statistical analysis was completed.
Statistical Methods
Data were analyzed using Stata (StataCorp. 2015. Stata Statistical Software: Release 14. College Station, TX: StataCorp LP) software. Due to the nonparametric nature of the data and small sample size, the Wilcoxon rank-sum test (Mann-Whitney) was used to compare the concentration of E. coli between terminal and tandem cleaning methods. CFU counts per area sampled were log10-transformed for consistency with published literature. To assess significance, an alpha of 0.05 was used. The lower hinge of the box-plots (Figs. 1–3) represents the 25th percentile of the data, whereas the upper hinge defines the 75th percentile of the distribution. To include data that were below the limit of detection (LOD) in the statistical analysis and box-plots, the equation was used to replace values below the LOD of 0.35 CFU/cm2 and nondetects.
RESULTS
Pretrial swabs showed no background contamination of tracer bacteria on sampled surfaces. Before cleaning, bacterial concentrations on seeded sites averaged 106 CFU during both terminal and tandem cleaning trials, with no statistically significant difference between the starting concentrations (p > 0.900). With all sample sites combined, manual terminal cleaning averaged a 2.4 log10 reduction compared with a 4.9 average log10 reduction (> 99.99% reduction in bacterial concentrations) with tandem cleaning (Fig. 1) (Table 1, Supplemental Digital Content 1, http://links.lww.com/CCX/A504). Thus, the tandem cleaning protocol increased cleaning efficacy by an average of approximately 2.5 log10 (p < 0.000).
Evaluation of individual surface efficacy showed improved reductions in bacterial concentrations for every site tested, including chairs, walls, floors, bedside tables, remote controls, bed rails, IV poles, under bed surfaces, sink faucets, bathroom faucets, toilet flush handles, shower handles, exit door handles, and bathroom doors. Statistically significant differences (p < 0.05) between terminal cleaning and tandem cleaning protocols were shown for chairs, walls, floors, remotes, IV poles, bathroom faucets, and bathroom doors (Fig. 2) (Table 2, Supplemental Digital Content 1, http://links.lww.com/CCX/A504). Tandem cleaning was most effective on walls, bed rails, toilet flush handles, shower handles, and bathroom doors where no bacterial tracer was detected in any of the replicate trials. Terminal cleaning alone never reached nondetectable tracer levels and often produced highly variable results over a wide range of log10 reduction efficacy.
Surfaces that presented the greatest challenge for terminal cleaning efficacy, as indicated by the highest log10 mean tracer concentration values, included the remotes, IV poles, exit door handles, under bed surfaces, bathroom faucets, and doors and exit door handles. IV poles were consistently contaminated with the highest level of bacteria after terminal cleaning alone. Tandem cleaning, however, reduced bacterial concentrations by nearly 3 log10 more compared with terminal cleaning. The greatest differences (> 3 log10 concentrations) between the terminal and tandem cleaning efficacy was seen with exit door handles and bathroom doors.
Tandem cleaning was also less variable compared with terminal cleaning. Sites with the highest terminal cleaning variability included under bed surfaces, toilet flush handles, bed rails, floors, bedside tables, and exit door handles (Fig. 2) (Table 2, Supplemental Digital Content 1, http://links.lww.com/CCX/A504).
Grouping surface categories by room zones provides another tool for analysis of terminal versus tandem cleaning. Figure 3 (Table 3, Supplemental Digital Content 1, http://links.lww.com/CCX/A504) show results of log10 bacterial concentrations for patient contact surfaces (bedside tables, remotes, bed rails, IV poles, under bed surfaces); patient and visitor contact surfaces (chairs, walls, floors, exit door handles); and bathroom and sink surfaces (sink faucets, bathroom faucets, toilet flush handles, shower handles, bathroom doors). When grouped by zone, there was a significant difference between terminal and tandem cleaning protocols for every group, with tandem cleaning achieving at least a 2 log10, and up to a 3.6 log10 (95th percentile), greater reduction in tracer concentrations (Fig. 3) (Table 3, Supplemental Digital Content 1, http://links.lww.com/CCX/A504).
The cross-contamination study aimed to evaluate the potential for the spread of bacterial tracer organisms from a single-seeded surface to nearby nonseeded surfaces and showed that terminal cleaning alone was more likely to spread viable tracers to other room sites. Cross-contamination among surfaces following terminal cleaning alone was documented in 50% of the total samples (n = 16). Sites most likely to be contaminated via cross-contamination during terminal cleaning were chair arms and floors. Tracer concentrations on cross-contaminated sites ranged from 1 to 3 log10. No evidence of cross-contamination was detected in any samples following tandem cleaning protocols.
In this study, the room was always free of gross debris; therefore, the typical tasks of trash removal and bed linen changes were omitted from both the manual and tandem cleaning protocols. The average EVS manual cleaning time for this terminal cleaning was 18.2 minutes compared with 6.5 minutes for the modified manual cleaning protocol. The tandem cleaning protocol resulted in a 64% improvement in manual labor efficiency.
DISCUSSION
This study provides a quantitative analysis of an abbreviated terminal cleaning in tandem with whole-room atomized disinfection compared with existing terminal cleaning protocols. Justification for improvement needs in environmental controls for pathogen transmission is readily apparent by the persistently high levels of HAIs that result in up to 720,000 infections, 75,000 deaths, and $30 billion in costs annually in the United States. (24,25). One in 25 patients will acquire an HAI in the United States, resulting in hospital stays extended by an average of 16.7 days and an average 23.6% higher likelihood of readmission within 30 days (24,26).
Fomites are commonly identified as sources of HAI transmission (2,5,9). Environmental surfaces close to the patient (e.g., bed rails and bedside tables) are more heavily contaminated and more frequently touched than other surfaces (e.g., floors) and thus may contribute to more environmental cross-contamination compared with other fomites (27–29). Also, soft surfaces such as curtains, fabric chairs, and linens have been linked to healthcare disease outbreaks and pose additional challenges for surface disinfectants (30–32). This study and others have shown a wide range of surface cleaning and disinfection efficacy in healthcare environments related to surface type and location, product ease of use and contact times, workflow challenges, EVS personnel behaviors and practices, and more (12,16). Evidence is growing in support of whole-room or no-touch methods to enhance terminal cleaning, (17,18,33,34) Others have shown that essential components of effective surface disinfection include the product selection and the practice of application (35). The two most studied no-touch technologies include ultraviolet devices and hydrogen peroxide vapors, both of which have demonstrated significant reductions with a broad array of HAI pathogens, including the highly resistant Clostridium difficile spores. An important consideration for some methods is not only efficacy but also the time required for room turnover. In terms of practice, each method has unique challenges. For example, methods using hydrogen peroxide vapors have been found to effectively reduce nosocomial vancomycin-resistant enterococci rates by 75% but may require 2–6 hours to complete, limiting its usefulness for routine application (36). Ultraviolet light systems also provide a high level of decontamination but are challenged by surfaces that are not in the line-of-sight (36). In this preliminary study, the tandem protocol used a whole-room HOCl atomizing disinfection system formulated to release 850–1,000 ppm of free chlorine at a pH of 6.0–6.5 to minimize corrosivity. The method resulted in up to a 6 log10 microbial reduction efficacy in less than 50 minutes of room turnover time; however, this system has not been evaluated against, nor is it generalizable to, a broad spectrum of pathogens, such as human viruses or C. difficile spores. Such an assessment is necessary to compare no-touch technology products and practices in the future.
Reducing HAI rates has the added benefit of improving antibiotic stewardship. Treating HAIs requires increased reliance on antibiotics that contributes to increases in antibiotic resistance, a recognized global health crisis (37). New approaches are needed to achieve increased efficacy for minimizing environmental contamination as one of the primary routes of HAIs. According to the Centers for Disease Control and Prevention’s National Healthcare Safety Network, hospital-onset methicillin-resistant Staphylococcus aureus (MRSA) baseline rates were reduced by 6% from 2015 to 2016. The U.S. Department of Health and Human Services, however, set a national target to reduce hospital-onset MRSA and other nosocomial infections by 50% (38). Two of the top five identified factors increasing the risk of HAIs included “healthcare settings that are not properly cleaned and disinfected” and “communicable diseases passing between patients and healthcare workers.” Meeting such ambitious targets will require a paradigm shift in healthcare hygiene goals to better address fomite controls.
Currently, there are no standards for healthcare environments related to bacterial contamination levels on surfaces and no defined risk targets (39). Although this study is limited to a single site, cleaning protocol, and vegetative bacterial surrogate, these preliminary results could be used to plan future studies to relate the efficacy of multiple terminal cleaning methods, alone and in tandem, to the expected reduction of microbial pathogens in hospital patient rooms. Although more information is needed to evaluate additional HAI pathogens, data from this study can also be used to inform quantitative exposure and risk assessment models for determining the relative bacterial risk reductions in patient populations following each cleaning application and levels of disinfection needed to meet related risk targets (39,40). This study highlights the importance of industry, academia, and healthcare partnerships to evaluate disinfection protocols and raise the standard of care. To address the limitations of this study, additional next steps are aimed at the evaluation of this tandem cleaning protocol in multiple sites with active patient care, additional pathogen types, and a wider variety of practice conditions.
CONCLUSIONS
Quantitative analysis shows that the addition of whole-room atomized disinfection to existing terminal cleaning protocols lowered manual labor times, improved disinfection outcomes, and eliminated the transfer of bacterial tracers to previously uncontaminated surfaces within patient rooms. Although this work is preliminary in nature, it provides the bases for an expanded study design to evaluate product efficacy with a wider variety of HAI pathogens and site scenarios. Persistent HAI infections suggest a need for increased efficacy in environmental hygiene; however, more research is required to evaluate necessary disinfection targets for achieving specific human health outcomes.
ACKNOWLEDGMENTS
We extend a special thanks to the study site personnel for their cooperation in the study and to Dr. Robert Canales for providing mentorship for statistical analysis.